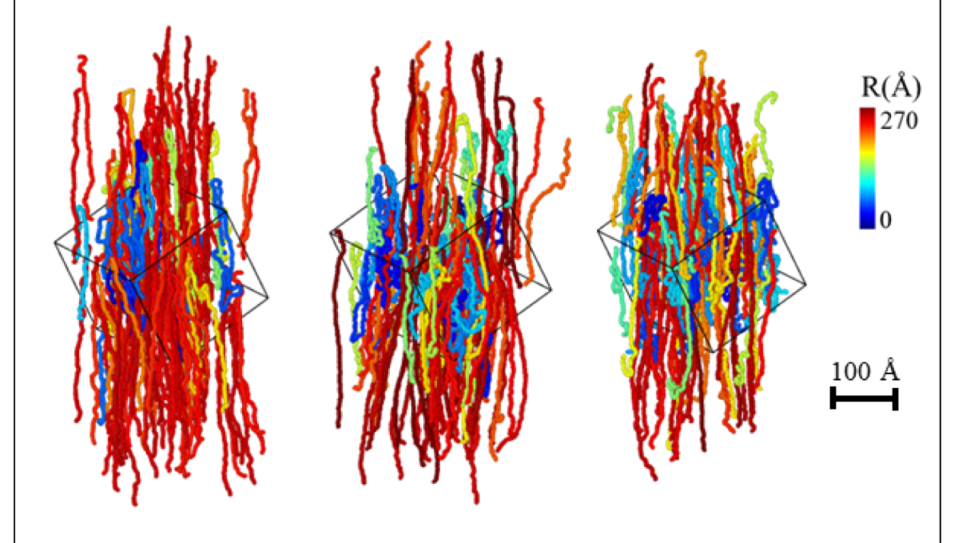
Visualization of the polymer backbone of polystyrene sulfonate with sulfonation fraction f after an elongation strain ε = 10 for strain rate ε̇ = 108 s −1 . Chains are colored according to their end-to-end distance R. Credit: S. S. Mohottalalage et al., Macromolecules 56, 947 (2023)