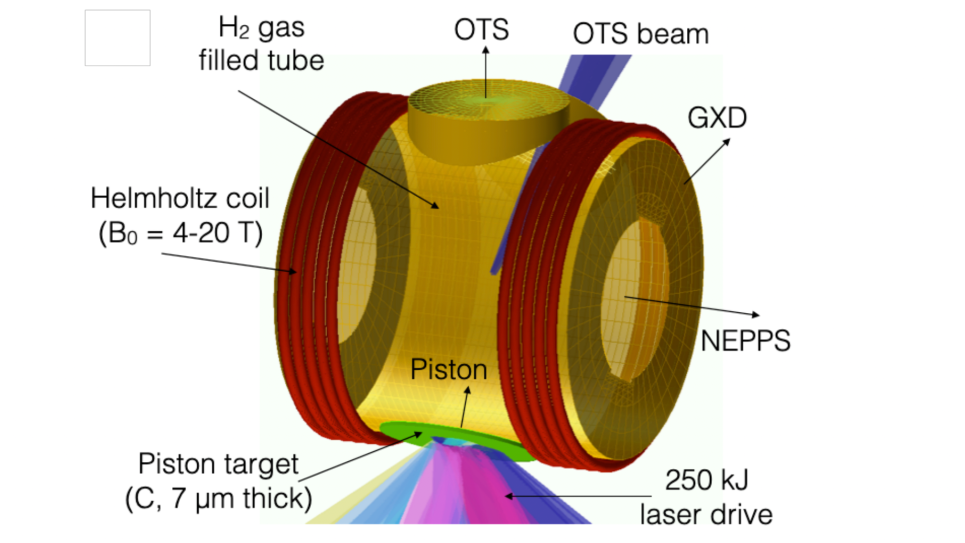
Schematic of the experimental configuration for the study of particle acceleration in magnetized shocks at NIF. Laser irradiation of a carbon foil drives a piston into a magnetized hydrogen plasma. Optical Thomson Scattering (OTS), Xray imaging (GXD) and particle spectrometers (NEPPS) are used to characterize the shock structure and particle acceleration for variable magnetizations and inclinations of the piston flow with respect to the ambient magnetic field.