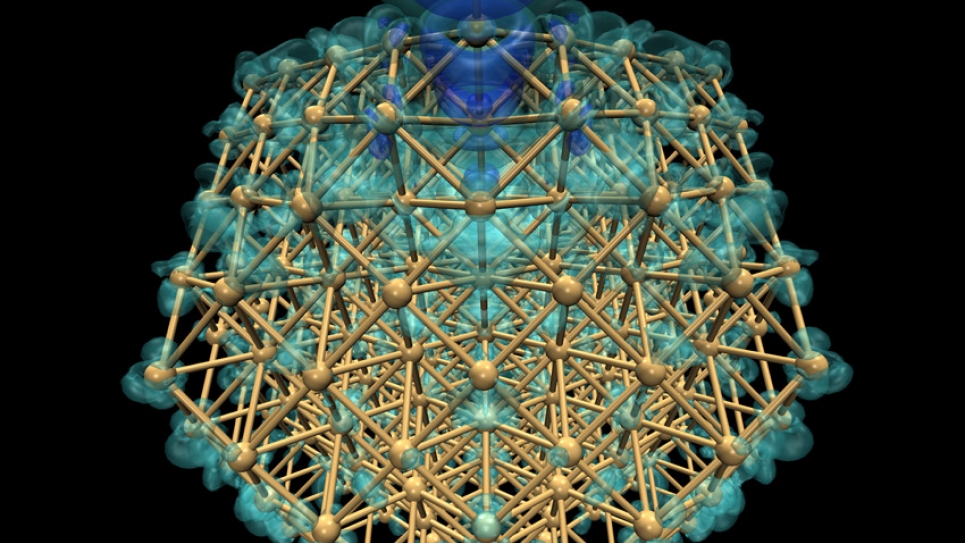
The proposed research will leverage new, highly parallelizable electronic structure (Density Functional Theory - DFT) codes and the computational resources available through the ALCC program to determine critical aspects of the stability and durability of electrocatalytic particles in the “non-scalable nano regime.” Such properties have not previously been studied in detail because, by their very nature, the properties of nanosized structures in this size range cannot be extrapolated from the corresponding properties of either molecules (or, equivalently, small clusters) or bulk matter. It is relatively straightforward to probe either of these size extremes with standard DFT calculations, but intermediate regimes – with metallic or oxidized particles in the size range of ~2-4 nm – can only be described by explicit DFT calculations on massively parallel leadership-class computing resources. Our study will thus be one of the first examples of the use of rigorous electronic structure calculations to determine the properties of metallic and partially oxidized particles across the size range at which such properties cannot be extrapolated from the bulk. We note that this study is distinct from the active INCITE proposal of the PI. The INCITE work, while also concerned with metallic nanoparticles, is exclusively focused on their catalytic properties for carbon monoxide oxidation and does not address their stability in electrochemical environments, which is the focus of the present work.
In addition to providing important fundamental insight into the nanoscale size-dependent properties of electrocatalyst nanoparticles, the proposed work will also address critical questions concerning the durability and degradation of nano-sized fuel cell electrocatalysts. Catalytic fuel cell electrodes, generally composed of platinum or platinum alloy nanoparticles, are often very efficient in the chemical reactions that they carry out. However, they also suffer from significant performance losses due to long- term instabilities, platinum loss, and degradation in harsh electrochemical environments.
In spite of the critical technological importance of these degradative processes, though, many fundamental atomic-scale mechanisms of how the degradation occurs are not understood. In the proposed work, we will analyze such mechanisms for the competition between electrochemical metal dissolution and surface passivation via oxide formation.
The competition between these effects is key to understanding nanoparticle durability in electrochemical environments and is likely to be strongly particle size dependent; indeed, the effects are thought to change significantly in the critical size range of 3-4nm –precisely the non-scalable range that our calculations are capable of rigorously treating.
The calculations will be performed on Argonne’s Blue Gene/P system using GPAW, a grid-based projector augmented wave DFT code from Jens Nørskov’s group. GPAW currently scales to 20% of Intrepid, 8-racks ~ 32,000 computational cores, and is reliably able to calculate energetics for metal and oxide nanoparticles with up to 15,000 valence electrons. GPAW is presently used in a number of INCITE and ALCC allocation at the Argonne Leadership Computing Facility (ALCF). Additionally, GPAW is the primary application in a Blue Gene/Q Early Science Program allocation.
As a secondary goal of our study, we will also perform classical molecular dynamics (CMD) simulations to treat oxidation and dissolution of platinum nanoparticles with diameters of tens of nanometers (millions of atoms). This “large-particle limit” will provide an important boundary condition for our DFT analyses of smaller particles.